Palaeognathae
John Harshman and Joseph W. Brown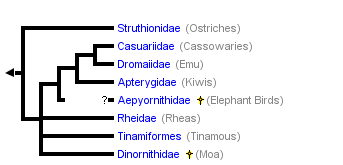


This tree diagram shows the relationships between several groups of organisms.
The root of the current tree connects the organisms featured in this tree to their containing group and the rest of the Tree of Life. The basal branching point in the tree represents the ancestor of the other groups in the tree. This ancestor diversified over time into several descendent subgroups, which are represented as internal nodes and terminal taxa to the right.
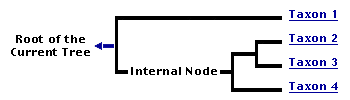
You can click on the root to travel down the Tree of Life all the way to the root of all Life, and you can click on the names of descendent subgroups to travel up the Tree of Life all the way to individual species.
For more information on ToL tree formatting, please see Interpreting the Tree or Classification. To learn more about phylogenetic trees, please visit our Phylogenetic Biology pages.
close boxDiscussion of Phylogenetic Relationships
Relationships among paleognaths have been controversial and are likely to remain so for some time, but the tree shown above is the current best estimate, based on analysis of 20 unlinked nuclear genes (Harshman et al. 2008). This tree shows the ratites (flightless paleognaths, including all extant paleognaths except the tinamous) to be polyphyletic, a major departure from most recent analyses of molecular (Cooper et al. 2001, Haddrath and Baker 2001, Sibley and Ahlquist 1990) and morphological (Lee et al. 1997, Livezey and Zusi 2007) data.All possible relationships among the paleognaths, including complete polyphyly, have been proposed at various times (Olson 1985, Houde 1988), but in the past few decades, a few points have been settled. Paleognath monophyly has become clear, and it is universally agreed that Dromaiidae (emus) and Casuaridae (cassowaries) are close relatives. And, until recently, monophyly of the ratites had been generally accepted. Beyond this, however, there has been no agreement. Most morphological analyses have found Apterygidae (kiwis) to be the sister group of all other ratites. Most molecular analyses have found kiwis to be the sister group of emus and cassowaries.
There have been a few holdouts against ratite monophyly; both Elzanowski (1995) and Bock and Buhler (1990), on the basis of morphological characters, found Struthionidae (ostriches) to be the sister group of all other paleognaths, and this is also what the nuclear DNA data show. Nuclear data also find, in accord with previous molecular analyses, that kiwis are the sister group of the emus and cassowaries, the three groups together consituting a clade of Australasian ratites. However, the data are unable to resolve relationships among the Australasian ratites, Rheidae (rheas), and tinamous.
The importance of evolutionary models in phylogenetic analyses is borne out by the most recent analyses of mitochondrial data (Phillips et al., 2010), in which the same data that previously resulted in ratite monophyly have been reanalyzed using a more complex model, with results consistent with the nuclear DNA tree above; that is, with ostrich as the sister group of all other paleognaths, though support is poor.
This tree implies that the various flightless paleognaths lost their ability to fly (and thus their sternal keels) independently of each other. The alternative possibility, that tinamous regained the ability to fly (and their sternal keels) after inheriting flightlessness from a common ratite ancestor, is considered less likely.
Placement of the extinct groups is unclear. Morphological and mitochondrial DNA data are in disgreement on relationships of Dinornithidae (moas), and there are so far no nuclear DNA sequences for extinct birds. The recent reanalysis of mitochondrial data (Phillips et al., 2010) found moas to be the sister group of tinamous. This is also in agreement with a combined analysis of nuclear and mitochondrial data (Harshman, unpublished), in which moas were represented by mitochondrial data only.
For more information about alternative hypotheses see Paleognath Relationships.
References
Amadon, D. 1947. An estimated weight of the largest known bird. Condor 49: 159-164.
Ansari, H., N. Kakagi, and M. Sasaki. 1988. Morphological differentiation of sex chromosomes in three species of ratite birds. Cytogenetics and Cell Genetics 47: 185-188.
Baccetti, B., A. G. Burrini, and E. Falchetti. 1991. Spermatozoa and relationships in palaeognath birds. Biology of the Cell 71: 209-216.
Bock, W. J., and P. Bühler. 1990. The evolution and biogeographical history of the palaeognathous birds. Pages 31-36 in Proceedings of the international centennial meeting of the Deutsche Ornithologen-Gesellschaft (R. van den Elzen, K.-L. Schuchmann and K. Schmidt-Koenig, eds.). Verlag der Deutschen Ornithologen-Gesellschaft, Bonn.
de Boer, L. E. M. 1980. Do the chromosomes of the kiwi provide evidence for a monophyletic origin of the ratites? Nature 287: 84-85.
Caspers, G. J., J. Wattel, and W. W. de Jong. 1994. Alpha A-crystallin sequences group tinamou with ratites. Molecular Biology and Evolution 11: 711-713.
Cooper, A., C. Lalueza-Fox, S. Anderson, A. Rambaut, J. Austin, and R. Ward. 2001. Complete mitochondrial genome sequences of two extinct moas clarify ratite evolution. Nature 409: 704-707.
Cooper, A., C. Mourer-Chauviré, G. K. Chambers, A. von Haeseler, A. C. Wilson, and S. Pääbo. 1992. Independent origins of New Zealand moas and kiwis. Proceedings of the national Academy of Sciences of the USA 89: 8741-8744.
Cracraft, J. 1974. Phylogeny and evolution of the ratite birds. Ibis 116: 494-521.
Cubo, J. 2003. Evidence for speciational change in the evolution of ratites (Aves: Palaeognathae). Biological Journal of the Linnean Society 80: 99-106.
Davies, S. J. J. F. 1967. Sexual dimorphism in the emu. Emu 67: 23-26.
Davies, S. J. J. F. 2002. Ratites and Tinamous. Bird Families of the World. Oxford University Press, Oxford, New York.
Edwards, S. V., B. Fertil, A. Giron, and P. J. Deschavanne. 2002. A genomic schism in birds revealed by phylogenetic analysis of DNA strings. Systematic Biology 51: 599-613.
Elzanowski, A. 1995. Cretaceous birds and avian phylogeny. Courier Forschungsinstitut Senckenberg 181:37-53.
Freitag, S., and T. J. Robinson. 1993. Phylogeographic patterns in mitochondrial DNA of the ostrich (Struthio camelus). Auk 110: 614-622.
García-Moreno, J., and D. P. Mindell. 2000. Rooting a phylogeny with homologous genes on opposite sex chromosomes (gametologs): a case study using avian CHD. Molecular Biology and Evolution 17: 1826-1832.
García-Moreno, J., M. D. Sorenson, and D. P. Mindell. 2003. Congruent avian phylogenies inferred from mitochondrial and nuclear DNA sequences. Journal of Molecular Evolution 57: 27-37.
Härlid, A., A. Janke, and U. Arnason. 1997. The mtDNA sequence of the ostrich and the divergence between Paleognathous and Neognathous birds. Molecular Biology and Evolution 14: 754-761.
Haddrath, O., and A. J. Baker. 2001. Complete mitochondrial genome sequences of extinct birds: ratite phylogenetics and the vicariance biogeography hypothesis. Proceedings of the Royal Society of London Biological Sciences 268: 939-945.
Harrison, G. L., McLenachan, M. J. Phillips, K. E. Slack, A. Cooper, and D. Penny. 2004. Four new avian mitochondrial genomes help get to basic evolutionary questions in the late Cretaceous. Molecular Biology and Evolution 21: 974-983.
Harshman, J., E. L. Braun, M. J. Braun, C. J. Huddleston, R. C. K. Bowie, J. L. Chojnowski, S. J. Hackett, K.-L. Han, R. T. Kimball, B. D. Marks, K. J. Miglia, W. S. Moore, S. Reddy, F. H. Sheldon, D. W. Steadman, S. J. Steppan, C. C. Witt, and T. Yuri. 2008. Phylogenomic evidence for multiple losses of flight in ratite birds. Proc. Natl. Acad. Sci. USA 105:13462-12467.
Houde, P. 1988. Paleognathous birds from the early Tertiary of the northern hemisphere, 22. Nuttall Ornithological Club, Cambridge, Mass.
Huyen, L., C. D. Millar, and D. M. Lambert. 2002. A DNA test to sex ratite birds. Molecular Ecology 11: 851-856.. Nature 425: 175-178.
Jamieson, B. G. M. 2007. Avian spermatozoa: Structure and phylogeny. Pages 349-512 in B. G. M. Jamieson (ed.), Reproductive biology and phylogeny of birds, Science Publishers, Enfield, NH.
Lee, K., J. Feinstein, and J. Cracraft. 1997. The phylogeny of ratite birds: resolving conflicts between molecular and morphological data sets. Pp 173-208 in D. P. Mindell (ed.), Avian Molecular Evolution and Systematics. Academic Press, San Diego.
Livezey, B. C., and R. L. Zusi. 2007. Higher-order phylogeny of modern birds (Theropoda, Aves: Neornithes) based on comparative anatomy. II. Analysis and discussion. Zool. J. Linn. Soc. 149:1-95.
Mayr, G., and J. Clarke. 2003. The deep divergences of neornithine birds: a phylogenetic analysis of morphological characters. Cladistics 19: 527-553.
Osuga, D. T., and R. E. Feeney. 1968. Biochemistry of egg-white proteins of ratite group. Archives of Biochemistry and Biophysics 124: 560-574.
Parkse, K. C., and G. A. Clark Jr. 1966. An additional character linking ratites and tinamous, and an interpretation of their monophyly. Condor 68: 459-471.
Phillips, M. J., G. C. Gibbs, E. A. Crimp, and D. Penny. 2010. Tinamous and moas flock together: Mitochondrial genome sequence analysis reveals independent losses of flight among ratites. Syst. Biol. 59:90-107.
Prager, E. M., A. C. Wilson, D. T. Osuga, and R. E. Feeney. 1976. Evolution of flightless land birds on southern continents: Transferrin comparison shows monophyletic origin of ratites. Journal of Molecular Evolution 8: 283-94.
Sibley, C. G., and J. A. Ahlquist. 1990. Phylogeny and classification of birds, Yale U. Press, New Haven.
Sibley, C. G., and C. Frelin. 1972. Egg-white protein evidence for ratite affinities. Ibis 114: 377-387.
Silynroberts, H., and R. M. Sharp. 1985. Preferred orientation if calcite in the ratite and tinamou eggshells. Journal of Zoology 205: 39-52.
Silynroberts, H., and R. M. Sharp. 1986. Preferred orientation if calcite in the Aepyornis eggshell. Journal of Zoology 208: 475-478.
Stapel, S. O., J. A. Leunissen, M. Versteeg, J. Wattel, and W. W. de Jong. 1984. Ratites as oldest offshoot of avian stem - evidence from alpha-crystallin A sequences. Nature 311: 257-259.
Tang, B., Y. H. Huang, L. Lin, X. X. Hu, J. Dong, P. Yao, L. Zhang, and N. Li. 2003. Isolation and characterization of 70 novel microsatellite markers from ostrich (Struthio camelus) genome. Genome 46: 833-840.
van Tuinen, M. C. G. Sibley, and S. B. Hedges. 1998. Phylogeny and biogeography of ratite birds inferred from DNA sequences of the mitochondrial ribosomal genes. Molecular Biology and Evolution 15: 370-376.
van Tuinen, M. C. G. Sibley, and S. B. Hedges. 2000. The early history of modern birds inferred from DNA sequences of nuclear and mitochondrial ribosomal genes. Molecular Biology and Evolution 17: 451-457.
Weir, K. A., and C. A. Lunam. 2004. A histological study of emu (Dromaius novaehollandiae) skin. Journal of Zoology 264: 259-266.
Zelenitsky, D. K., and S. P. Modesto. 2003. New information on the eggshell of ratites (Aves) and its phylogenetic implications. Canadian Journal of Zoology 81: 962-970.
Title Illustrations

Scientific Name | Struthio camelus |
---|---|
Location | Simon's Town, Western Cape, South Africa |
Specimen Condition | Live Specimen |
Source | Wild Ostrich |
Source Collection | Flickr |
Image Use |
![]() |
Copyright | © 2005 Josh M |
Scientific Name | Dromaius novaehollandiae |
---|---|
Location | Captive at Colin Mackenzie Sanctuary, Healesville, South Australia |
Comments | Emus |
Creator | Dr. G Dallas and Margaret Hanna |
Specimen Condition | Live Specimen |
Source Collection | CalPhotos |
Copyright |
© 2004 California Academy of Sciences
![]() |
Scientific Name | Eudromia elegans |
---|---|
Location | Biedma, Chubut, Argentina |
Specimen Condition | Live Specimen |
Source | Elegant Crested Tinamou |
Source Collection | Flickr |
Image Use |
![]() |
Copyright |
©
![]() |
About This Page
Joseph W. Brown
University of Michigan Museum of Zoology, Ann Arbor, Michigan, USA
Correspondence regarding this page should be directed to John Harshman at
Page copyright © 2010 and Joseph W. Brown
Page: Tree of Life
Palaeognathae.
Authored by
John Harshman and Joseph W. Brown.
The TEXT of this page is licensed under the
Creative Commons Attribution-NonCommercial License - Version 3.0. Note that images and other media
featured on this page are each governed by their own license, and they may or may not be available
for reuse. Click on an image or a media link to access the media data window, which provides the
relevant licensing information. For the general terms and conditions of ToL material reuse and
redistribution, please see the Tree of Life Copyright
Policies.
- First online 14 December 2005
- Content changed 13 May 2010
Citing this page:
Harshman, John and Joseph W. Brown. 2010. Palaeognathae. Version 13 May 2010 (under construction). http://tolweb.org/Palaeognathae/15837/2010.05.13 in The Tree of Life Web Project, http://tolweb.org/